What matters besides light?
A Guide to Non-Photic Zeitgebers
We can all agree that the difference between night and day is, well, night and day when it comes to light. The progressive change in light present in our environment is subconsciously tracked by our bodies and that information is used to help time our sleep-wake cycles. Of course, not everyone has the physical ability to perceive the changes in light which occur over the course of the day. Yet some blind individuals are still able to entrain accurately to their environment without these crucial photic cues. How is that so?
Light is the strongest “zeitgeber”— or, environmental cue that provides input to the circadian clock. Our bodies use the signals from zeitgebers to try to synchronize our internal clocks with our environments. For example, the decrease of light over time that you experience while watching a sunset can communicate to your body that nighttime is approaching. If you keep the lights on instead, your body’s clock can be delayed and production of the hormone for night, melatonin, can be suppressed. Because of this, it’s important to think about what signal your light exposure is sending to your internal clock (cough, screens at night, cough). But light is not the only player in town. There are other zeitgebers besides light that influence your circadian time.
Before getting into the weeds, it will help to have a mental map for how your body’s clocks are organized. Our circadian clocks are composed of a central clock and peripheral clocks. The central clock acts as the command center located in the suprachiasmatic nucleus (SCN), sending signals to the various tissue-specific peripheral clocks spread throughout the body. The phase of each peripheral clock is influenced by both the central clock and factors specific to that system, allowing different biological functions to be coordinated through the central clock while being autonomous enough to respond to stimuli specific to that system. For example, as we’ll discuss below, the metabolic clock is driven by both the central clock but is also affected directly by meal timing. Some signals may primarily affect peripheral rhythms, while others can affect both peripheral and central rhythms.
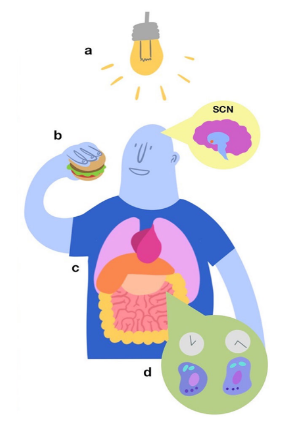
Before we talk about these non-light inputs to your body’s time, let’s talk about phase-response curves. The standard way to track how a zeitgeber advances or delays your central circadian pacemaker is through phase-response curves (PRCs). These graphs represent the timing of a zeitgeber stimulus (x-axis) and its quantitative effect on the timing of a circadian biomarker (y-axis), like shifts in melatonin timing or core body temperature minimum. A phase-response curve can tell us when a zeitgeber will advance you, when it will delay you, and when it will have essentially no effect.
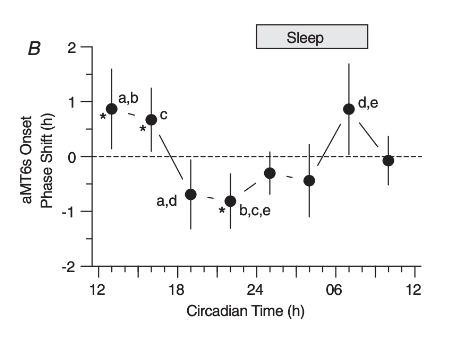
Exercise has been shown to induce central clock phase changes dependent upon the timing of activity. One study in particular, which produced the PRC above, recruited 101 physically active adults to investigate the effects of exercise on circadian phase. Participants were put on a 90 minute ultradian light schedule (60 minutes light/wake followed by 30 minutes dark/sleep) for approximately 6 days. Baseline aMT6s (urinary melatonin) measurements were made for individuals and averaged across the whole sample of participants. The difference between the individual and sample phase was determined and then subtracted from the external clock time of exercise to calculate a “circadian time” adjusted for interindividual differences.
Each participant performed 1 hour of moderate treadmill exercise at the same clock time for 3 consecutives days following baseline, isolating the effects of exercise on phase. Moderate exercise was quantified as 65-75% of the heart rate reserve calculated for each participant based on their individual maximal heart rate. This study concluded that exercise at 7am and between 1pm and 4pm causes phase advances to aMT6s timing, whereas exercise between 7pm and 10pm provoked phase delays.
These results can be read from the PRC above by identifying the selected time along the x-axis and reading the corresponding point’s phase shift, quantified by the y-axis. For example, exercising at 7pm (19 h) results in approximately -0.75 h phase shift. The negative value indicates a delay, whereas a positive would indicate an advance. Thus, exercise at 7pm causes a little less than an hour phase delay based on the above PRC.
A similar pattern of phase advance and delay regions can be observed in the human response to light, especially in studies with parallel protocols or similar duration of stimulus. Intuitively, the analogous behavior of morning advances and evening delays produced by light and physical activity makes sense—light and activity are often correlated, so if they told really different circadian stories, it would be pretty strange. The authors of the exercise PRC research note that the phase shifting strength of exercise is comparable to that of light exposure, making exercise another great tool for your circadian management tool belt.
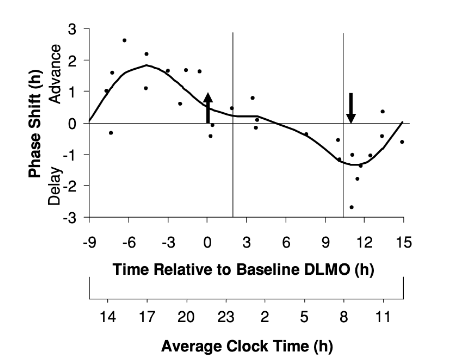
Oral melatonin supplements have also been shown to shift central clock outputs like dim light melatonin onset. In general, melatonin dosing tends to do the opposite of what light exposure would do at the same time—delaying you when you’d be advanced by light, or advancing you when you’d be delayed by light. While most people don’t think of melatonin as something that can be mistimed, the phase-response curve tells us that melatonin at the wrong time might have no phase shift whatsoever, or could delay you when you’d really prefer to be advanced (e.g. if you want to fall asleep faster at night).
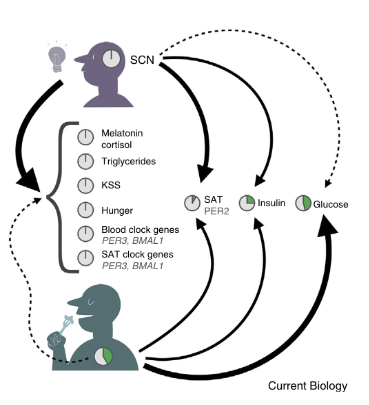
Eating patterns are interesting. When you keep light exposure patterns fixed and change the timing of meals, the vast majority of relevant outputs—melatonin, cortisol, hunger, triglycerides, and genes like PER3 and BMAL1—stick with the patterns set by light (in other words, they follow the SCN). A few of the players track with meal timing, however: glucose, and, to a lesser extent, PER2 and insulin patterns, showed significant phase shifts in response to the food time changing.
This means that misaligning your meal timing with your light timing could result in a kind of desynchrony, in which your light and your food are sending two different time-keeping signals, and in turn throwing your metabolic processes out of whack. This desynchrony—or, specifically, avoiding this desynchrony—could be the reason why time-restricted eating (TRE) has been found to improve cardiometabolic health.
So light’s not the whole story. On the one hand, that means there are more circadian-relevant factors to have to worry about; on the other, that means we have more knobs to turn as we try to help people achieve optimal circadian health. Can’t get bright light? Why not try exercise instead? Need more than just a light dose? Here’s the right time for melatonin, for you. At Arcascope, we want to use all the tools available to us to help you optimize your external and internal cues, in search of synchronous bliss.
This blog post was written by Carrie Fulton, one of Arcascope’s interns. Thanks, Carrie, for your hard work!
References
- St Hilaire MA, Klerman EB, Khalsa SB, Wright KP Jr, Czeisler CA, Kronauer RE. Addition of a non-photic component to a light-based mathematical model of the human circadian pacemaker. J Theor Biol. 2007 Aug 21;247(4):583-99. doi: 10.1016/j.jtbi.2007.04.001. Epub 2007 Apr 4. PMID: 17531270; PMCID: PMC3123888.
- Celine Vetter, Frank A.J.L. Scheer, Circadian Biology: Uncoupling Human Body Clocks by Food Timing, Current Biology, Volume 27, Issue 13, 2017, Pages R656-R658, ISSN 0960-9822, https://doi.org/10.1016/j.cub.2017.05.057. (https://www.sciencedirect.com/science/article/pii/S0960982217306231)
- Youngstedt SD, Elliott JA, Kripke DF. Human circadian phase-response curves for exercise. J Physiol. 2019 Apr;597(8):2253-2268. doi: 10.1113/JP276943. Epub 2019 Mar 18. PMID: 30784068; PMCID: PMC6462487.
- Burgess HJ, Revell VL, Molina TA, Eastman CI. Human phase response curves to three days of daily melatonin: 0.5 mg versus 3.0 mg. J Clin Endocrinol Metab. 2010 Jul;95(7):3325-31. doi: 10.1210/jc.2009-2590. Epub 2010 Apr 21. PMID: 20410229; PMCID: PMC2928909.
- Burgess HJ, Revell VL, Eastman CI. A three pulse phase response curve to three milligrams of melatonin in humans. J Physiol. 2008 Jan 15;586(2):639-47. doi: 10.1113/jphysiol.2007.143180. Epub 2007 Nov 15. Erratum in: J Physiol. 2008 Mar 15;586(6):1777. PMID: 18006583; PMCID: PMC2375577.
- Mohawk JA, Green CB, Takahashi JS. Central and peripheral circadian clocks in mammals. Annual review of neuroscience. 2012;35:445. doi:10.1146/annurev-neuro-060909-153128.
- Wehrens SM, Christou S, Isherwood C, Middleton B, Gibbs MA, Archer SN, Skene DJ, Johnston JD. Meal timing regulates the human circadian system. Current Biology. 2017 Jun 19;27(12):1768-75.
- St Hilaire, M.A., Gooley, J.J., Khalsa, S.B.S., Kronauer, R.E., Czeisler, C.A. and Lockley, S.W. (2012), Human phase response curve to a 1 h pulse of bright white light. The Journal of Physiology, 590: 3035-3045. https://doi.org/10.1113/jphysiol.2012.227892
- Kripke, D.F., Elliott, J.A., Youngstedt, S.D. et al. Circadian phase response curves to light in older and young women and men. J Circad Rhythms 5, 4 (2007). https://doi.org/10.1186/1740-3391-5-4